Overview of Technical Statement and New Challenges
September 30, 2023 - By Hedda Grelz, Andres Calderon, Oselumenosen Ekhuemelo, and Adolfo Diaz
Here is the second update on Team Navy’s Capstone Project: An SMA-actuated safety vent for lithium-ion battery packs. The team has made revisions to the key physical constraints presented in the first blog post after more literature review and feedback from capstone instructors and the project mentor. The SMA transition temperature for the opening of the vent has been adjusted to 70 °C. LIB cells ignite at 120-130°C during thermal runaway, but the air around the cell will not reach such high temperatures as fast [1]. Therefore, the transition temperature for the vent has to be lower. Because of hysteresis in the SMA, the closing temperature (reverse transition) will happen at a lower temperature. The team has set a goal to have the vent close when the battery has cooled down to 60 °C [2]. The vent will be designed for an electric car battery. The team does not plan to test the vent with a real lithium-ion battery for this project since it poses safety and budget challenges. The team will instead design a simple casing to act as the battery pack and use a hot air blower to imitate thermal runaway. For dimensional references, the team intends to use Tesla’s small battery which has the size of 68.5 x 30 x 75 cm (length x width x height) to create the testing chamber [3].
One of the main advantages of the team’s vent design is that it enables active opening by including a control system that can provide joule heating of the SMA and therefore activation at lower temperatures. This creates an opportunity for the vent to be connected to for example vehicle collision systems, which means that the vent could be opened preventively when a bad car crash has happened and the battery safety is in danger. The control system is a crucial part of the design and the team has identified three main physical constraints for this system: voltage requirement, operational temperature, and temperature sensor and feedback accuracy. The numbers are presented in Table 1.
The time from the initial battery defect to ignition in the event of thermal runaway has been reported to be 100 seconds. However, the team will set goals for significantly faster operation times from earlier prevention of thermal runaway. The constraint for the opening time, both passive and active, has been set to 10 seconds. Releasing gases any faster than this might cause the gas to escape rapidly, potentially causing large explosions [1]. The battery consists of highly flammable organic solvents and also can contain explosive materials such as perchlorates [4]. Due to this composition, the battery vent system should be able to close fully after venting to prevent the battery from extended exposure to the outside environment, which can result in triggering reactions in these components and worsening the effects of thermal runaway. The team shall address this limitation by making the battery closing time 1 min. The major physical constraints are summarized in Table 1.
Table 1: Table of Major Project Constraints. To be used to evaluate if the device meets the minimum requirements for a successful solution.
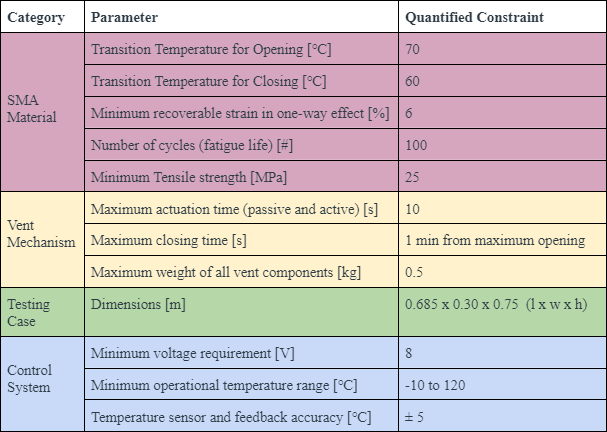
The main physical constraints that are expected to pose the biggest challenges in our design are the SMA transition temperature, the maximum opening and closing times, and the temperature sensor - feedback accuracy. SMAs don’t have an exact transition temperature, rather it is a temperature window in which the transition occurs. This is due to the hysteresis phenomenon of the SMA, which is due to the strain incompatibility that introduces several irreversible processes in the transformation between phases, such as the generation of dislocations and microcracks. The thermal hysteresis of SMAs is demonstrated in Figure 1 which shows the martensite composition based on temperature. The SMA will have one transition temperature for the heating, but because of hysteresis, the transition temperature for the cooling will be lower. The team has set the goal to have a hysteresis window around 10 [℃] so the vent will open at 70 [℃] and close at 60 [℃]. The amount of thermal hysteresis in an SMA depends on several different factors including alloy composition, heat treatment, and thermal loading in each cycle [2]. This adds another dimension to the selection of the SMA material and its heat treatment. The team plans to in an early stage of the project test the SMA hysteresis empirically, with both joule heating and passive heating, once the material is selected to verify that it falls within the desired range.

Figure 1: SMA thermal hysteresis [5]
The opening and closing times will depend on several different factors. The opening is not expected to pose significant challenges, but the closing will. Slow cooling time is one of the main drawbacks of SMA actuators and is therefore a krewe issue to address in this project. Cooling time depends mainly on the dimensions of the actuator and alloy composition [6]. It can be as fast as 4-5 seconds but can take longer if the ambient temperature increases slowly. The team will therefore attempt to keep the SMA wire diameter as small as possible for optimal cooling, and keep the actuator in a casing to prevent excessive exposure to the higher temperatures in the battery casing. The team knows that there might potentially have to be a cooling component added to speed up the cooling process of the SMA, but the team will first attempt using natural cooling since the closing time doesn’t need to be very fast.
Lastly, the team anticipates that the feedback and temperature sensor accuracy will pose challenges to the design. The SMA will be used as both an actuator and a sensor, and therefore the hysteresis of the SMA plays an important role in the design of the control system’s sensor-feedback system as well. This will be addressed through careful control system modeling and design with an emphasis on reviewing literature related to SMA control. It is common to use pulse-width modulation (PWM) and a Proportional–integral–derivative controller (PID controller) for SMA applications. The team will therefore start with investigating these options [7, 8].
The main technical analyses that the team will conduct to address the physical constraints and optimize the performance of the vent design are SMA material analysis design and testing, two different types of Finite Element Analysis (FEA), and a control system modeling and design. The SMA material analysis will include investigating how the SMA composition and wire thickness will affect transition temperature, maximum strain, hysteresis, and cooling time. Once the SMA is selected, it will be empirically tested to determine the actual thermal hysteresis, transition temperature window, and maximum strain. The team will also conduct a thermal loading FEA on the selected SMA spring design. This will be done in a MATLAB script where stiffness matrices and constitutional equations will be set up and elemental analysis will be performed to evaluate how different thermal loads affect the deformation of the SMA. This is something that the team’s mentor, Dr. Gangbing Song will teach the team how to do it as it is a bit different from the traditional FEA that is conducted in software programs such as COMSOL and is based on mechanical loads. A regular SMA will also be conducted on the casing and closed vent to ensure that it can withstand the pressure build-up during thermal runaway. This will be performed after a hand-calculated load analysis has been conducted.
The team also needs to predict the reaction of the SMA when it senses a temperature change and the control system sends a corresponding actuation signal to heat up the SMA and induce deformation and vent opening. This is crucial to optimize the function of the active opening of the vent and ensure that the SMA doesn’t get overheated or damaged by the joule heating. This will be done by control system modeling and design. In this process, the team first needs to model the SMA behavior theoretically with a constitutional relationship. The constitutive relationship of SMA refers to the changing relationship between stress, strain and temperature during phase transformation. It can be difficult to establish because of the nonlinearities inherent in all SMAs [9]. However, there are many types of constitutive relationships already developed in the literature for SMA actuators and sensors, so the team will use one of these and establish which one is best for the vent application based on the literature review. The team also has to include a phase transformation kinetics model of the SMA to be able to predict its dynamic response. Once these theoretical models have been established, a MATLAB/Simulink model of the SMA spring actuator can be created. This model will be used to predict the output of the system based on input and will be iteratively used to make decisions about control parameters to optimize system performance. The team will establish a closed feedback loop to ensure that the correct amount of joule heating is provided to the SMA based on the current temperature in the battery chamber. The control system can also help limit the effect of the SMA thermal hysteresis.
As the project moves forward, soft challenges not included in the project constraints will arise and require modifications to the initial design concept. The initial design concept is presented in Figure 2. In this simple design, the vent mechanism consists of a plate, an SMA spring, and a regular spring. The regular spring will be selected such that it has a spring stiffness in between the SMA martensite spring stiffness and the SMA austenite phase spring stiffness. The composition of this regular spring is one of the main soft challenges. Once the SMA heats up, the SMA spring overcomes the regular spring so when it expands, the regular spring is compressed and the vent opens. Once the temperature is lowered and the SMA returns to the martensite phase, the regular spring will overcome its stiffness and compress the SMA spring to close the vent.
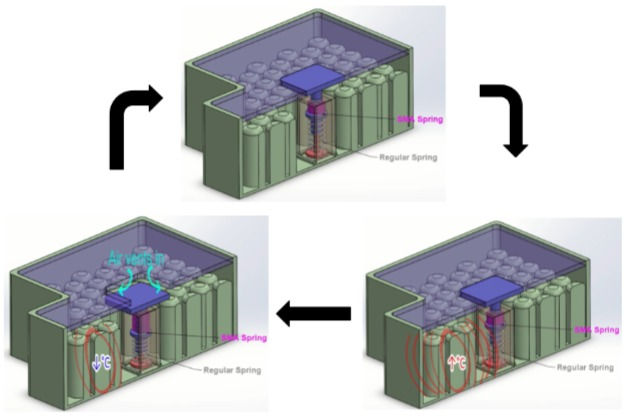
Figure 2: Three step SMA safety vent actuation and closing process
In certain cases, rapid release of internal gases may trigger combustion, thereby exacerbating the hazardous conditions we wish to minimize [10]. This composes a new soft challenge that the team had previously not envisioned. A large plate for the vent can result in too much gas release in a short amount of time. The team is currently considering a 3-stage opening vent to resolve this. The rate at which the air rushes in depends on a few factors like the pressure differential, size and shape of the opening, and obstructions. In this potential 3-stage vent, the first plate will open at 70 ℃. If the temperature in the battery is not lowered quickly, the SMA spring will keep extending to open a second and then a third plate to increase the pressure and temperature relief. Partial openings of the vent along with small holes on the sides of each stage will allow for slower venting, reducing the chance of combustion upon gas release. This is not a decision yet, but an important design consideration when developing the final CAD model in a couple of weeks.
Other soft challenges include material selection and ordering, and developing enough skills for MATLAB FEA modeling of the SMA and the control system modeling. The team is aware of the potential El Nino winter which could affect processing times when ordering materials that are needed. Therefore, the team will attempt to make these selections as soon as possible to ensure that all material comes in time for the prototyping stage of the project. As for the technical skills connected to the analysis, the team intends to start educational meetings with mentor Dr. Gangbing Song within the next two weeks to start learning the essential skills to develop these technical models.
That is all updates on the technical project plan for Team Navy’s SMA actuated safety vent for lithium-ion batteries. Feel free to contact our team with any additional questions!
References
[1] Wei, G., Huang, R., Zhang, G., Jiang, B., Zhu, J., Guo, Y., Han, G., Wei, X., and Dai, H., 2023, “A Comprehensive Insight into the Thermal Runaway Issues in the View of Lithium-Ion Battery Intrinsic Safety Performance and Venting Gas Explosion Hazards,” Applied Energy, 349, p. 121651.
[2] Nature, "Flexible and Transparent Electronic Devices Fabricated from Atomically Thin Two-Dimensional Nanomaterials," Scientific Reports, Vol. 6, Article No. 28244, 2016.
[3] “Electric Vehicle Conversion Using Tesla Batteries: Power Battery Blog: Power Battery.” Power Battery Blog | Power Battery, www.powerbattery.nl/resources/blog/electric-vehicle-conversion-using-tesla-batteries
[4] Lisbona, D., and Snee, T., 2011, “A Review of Hazards Associated with Primary Lithium and Lithium-Ion Batteries,” Process Safety and Environmental Protection, 89(6), pp. 434–442
[5] "Shape-memory alloy," Wikipedia, https://en.wikipedia.org/wiki/Shape-memory_alloy, Accessed September 30, 2023.
[6] Gupta, S., Sharma, S., & Gupta, T. K., "A Review on the Shape Memory Alloys," Materials Research Express, Vol. 7, No. 9, 092002, 2020.
[7] Junghyuk Ko, Martin B. Jun, Gabriele Gilardi, Edmund Haslam, and Edward J. Park. Fuzzy pwm-pid control of cocontracting antagonistic shape memory alloy muscle pairs in an artificial finger. Mechatronics, 21(7):1190–1202, 2011.
[8] Hao Yang, Min Xu, Weihua Li, and Shiwu Zhang. Design and implementation of a soft robotic arm driven by sma coils. IEEE Transactions on Industrial Electronics, 66(8):6108–6116, 2019.
[9] Bingshan, H., Fengchen, L., Binghao, M., Shiwei, C., Hongliu, Y., "Modeling and Positioning Control simulation Research on shape Memory Alloy spring Actuator," Micromachines, Vol. 13, No. 2, Article No. 178, 2022.
[10] Feng, X., Ren, D., He, X., and Ouyang, M., 2020, “Mitigating Thermal Runaway of Lithium-Ion
Batteries,” Joule, 4(4), pp. 743–770
Comentarios